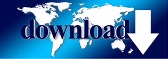

Sound passes through the system by compressing and expanding the springs, transmitting the acoustic energy to neighboring spheres. In real material terms, the spheres represent the material's molecules and the springs represent the bonds between them. The transmission of sound can be illustrated by using a model consisting of an array of spherical objects interconnected by springs. The distance was known by triangulation, and thus the speed that the sound had travelled was calculated. Measurements were made of gunshots from a number of local landmarks, including North Ockendon church. Laurence, Upminster to observe the flash of a distant shotgun being fired, and then measured the time until he heard the gunshot with a half-second pendulum. This is longer than the standard "international foot" in common use today, which was officially defined in 1959 as 304.8 mm, making the speed of sound at 20 ☌ (68 ☏) 1,055 Parisian feet per second).ĭerham used a telescope from the tower of the church of St. In 1709, the Reverend William Derham, Rector of Upminster, published a more accurate measure of the speed of sound, at 1,072 Parisian feet per second. ĭuring the 17th century there were several attempts to measure the speed of sound accurately, including attempts by Marin Mersenne in 1630 (1,380 Parisian feet per second), Pierre Gassendi in 1635 (1,473 Parisian feet per second) and Robert Boyle (1,125 Parisian feet per second). This error was later rectified by Laplace. The discrepancy is due primarily to neglecting the (then unknown) effect of rapidly-fluctuating temperature in a sound wave (in modern terms, sound wave compression and expansion of air is an adiabatic process, not an isothermal process). Sir Isaac Newton's 1687 Principia includes a computation of the speed of sound in air as 979 feet per second (298 m/s). Objects moving at speeds greater than the speed of sound ( Mach 1) are said to be traveling at supersonic speeds. The ratio of the speed of an object to the speed of sound (in the same medium) is called the object's Mach number. In fluid dynamics, the speed of sound in a fluid medium (gas or liquid) is used as a relative measure for the speed of an object moving through the medium. The speed of shear waves is determined only by the solid material's shear modulus and density.

The speed of compression waves in solids is determined by the medium's compressibility, shear modulus, and density. Shear waves in solids usually travel at different speeds than compression waves, as exhibited in seismology. Sound waves in solids are composed of compression waves (just as in gases and liquids) and a different type of sound wave called a shear wave, which occurs only in solids. In theory, the speed of sound is actually the speed of vibrations. In an exceptionally stiff material such as diamond, sound travels at 12,000 metres per second (39,000 ft/s), - about 35 times its speed in air and about the fastest it can travel under normal conditions. For example, while sound travels at 343 m/s in air, it travels at 1,481 m/s in water (almost 4.3 times as fast) and at 5,120 m/s in iron (almost 15 times as fast). However, the speed of sound varies from substance to substance: typically, sound travels most slowly in gases, faster in liquids, and fastest in solids. In colloquial speech, speed of sound refers to the speed of sound waves in air. The speed has a weak dependence on frequency and pressure in ordinary air, deviating slightly from ideal behavior. The speed of sound in an ideal gas depends only on its temperature and composition. More simply, the speed of sound is how fast vibrations travel. It depends strongly on temperature as well as the medium through which a sound wave is propagating. At 20 ☌ (68 ☏), the speed of sound in air is about 343 metres per second (1,125 ft/s 1,235 km/h 767 mph 667 kn), or one kilometre in 2.91 s or one mile in 4.69 s. The speed of sound is the distance travelled per unit of time by a sound wave as it propagates through an elastic medium.
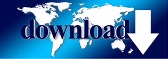